What Kinds Of Animals Are Most Efficient To Eat
Abstract
Purpose
Livestock already use almost global agronomical land, whereas the demand for animal-source food (ASF) is expected to increment. To address the contribution of livestock to global food supply, we need a mensurate for land use efficiency of livestock systems.
Methods
Existing measures capture different aspects of the debate about land utilize efficiency of livestock systems, such as plant productivity and the efficiency of converting feed, specially homo-inedible feed, into fauna products. So far, the suitability of land for cultivation of food crops has non been accounted for. Our land use ratio (LUR) includes all above-mentioned aspects and yields a realistic insight into country employ efficiency of livestock systems. LUR is divers as the maximum amount of human-digestible protein (HDP) derived from food crops on all land used to cultivate feed required to produce 1 kg ASF over the amount of HDP in that ane kg ASF. We illustrated our concept for three example systems.
Results and word
The LUR for the example of laying hens equaled ii.08, implying that land required to produce 1 kg HDP from laying hens could directly yield 2.08 kg HDP from human food crops. For dairy cows, the LUR was 2.10 when kept on sandy soils and 0.67 when kept on peat soils. The LUR for dairy cows on peat soils was lower compared to cows on sandy soils because state used to grow grass and grass silage for cows on peats was unsuitable for direct product of food crops. A LUR <1.0 is considered efficient in terms of global nutrient supply and implies that animals produce more HDP per square metre than crops.
Conclusions
Values <ane.0 demonstrate that livestock produce HDP more efficiently than crops. Such livestock systems (with a LUR < 1.0), therefore, do accept a role in future food supply and therefore contribute to food security. Our LUR offers identification of livestock production systems that contribute to global food supply, i.due east. systems that value country with depression opportunity costs for arable production and/or past-products from crop cultivation or the food or free energy industry.
Introduction
A growing and wealthier human population implies an increase in need for their needs, such as housing, infrastructure, energy and food, specially animal-source nutrient (ASF). The electric current livestock sector already uses about seventy % of global agronomical land (FAO 2009). The expected increase in need for ASF, therefore, volition further intensify global pressure on state. An increased pressure on land amplifies the risk of converting forests, wetlands or natural grasslands into agricultural country, resulting in emission of greenhouse gases and the loss of biodiversity and other important ecosystem services (Godfray et al. 2010; Foley et al. 2011). To limit state conversion, it is essential to eastward.g. improve land use efficiency of livestock systems.
It is generally acknowledged that increasing yields on existing land is cardinal to improve country employ efficiency in agriculture (Godfray et al. 2010; Tilman et al. 2011). Similarly, country use efficiency of livestock systems will meliorate with increasing yields of grazed pastures and feed crops per hectare. Land use efficiency of livestock systems, furthermore, tin improve by increasing feed efficiency, i.e. the efficiency of converting feed into ASF (De Vries and De Boer 2010). Also increasing crop or animal productivity, land use efficiency of livestock systems improves also by increasing the efficiency along the unabridged food chain, from "field-to-fork", implying a reduction in, for example, grazing losses, losses while storing feed crops, or losses while consuming ASF.
Information technology is increasingly recognized that, to reach hereafter food security, nosotros might meliorate not utilize highly productive croplands to produce feed for livestock. No matter how efficiently produced, direct consumption of cereals past humans is ecologically more efficient than consumption of ASF produced by animals fed with these cereals (Godfray et al. 2010; Foley et al. 2011). Improving country use efficiency of livestock systems, therefore, also implies feeding livestock mainly by-products from arable product or the food processing industry, that are non edible for humans; or grazing of livestock on "marginal land", i.eastward. state with low opportunity costs for arable production (Garnett 2009; Eisler et al. 2014).
All to a higher place-described aspects are essential to improve country use efficiency of livestock systems to increase nutrient supply and therefore contribute to food security. The urgent question remains, nevertheless, how land efficient are various livestock systems in terms of food supply? In this paper, we depict a novel method to calculate land employ efficiency of livestock systems, which enables identification and improvement of systems that do have a function in future food supply. To illustrate our concept, we computed our novel method for 3 case systems in kingdom of the netherlands: production of ASF (eggs and meat) from laying hens and production of ASF from dairy cows (milk and meat from the dairy subcontract) on peat soils and on sandy soils.
To demonstrate the importance of our novel concept, nosotros compared our findings with existing measures for land use efficiency. We, therefore, first describe in more detail how country use efficiency of livestock systems has developed and was measured to date.
Methods
Electric current drivers and existing measures of land use efficiency of livestock systems
In the by, improving country use efficiency of livestock systems was mainly driven by economical incentives and was directed at increasing productivity per hectare of land. Consequently, crop and animal productivity per hectare has increased enormously. For well-nigh cereal crops in the globe, yields have increased nearly linearly since 1960. Boilerplate US maize product, for example, increased 114 kg per hectare per year betwixt 1960 and 2011 (Grassini et al. 2013). Similarly, feed efficiency of livestock has improved continuously. The feed conversion ratio (FCR) of broilers (i.e. kg feed used per kg of final body weight), for example, was estimated to reduce by 0.02 kg feed/kg broiler meat per yr between 1960 and 2013 (Neeteson-van Nieuwenhoven et al. 2013).
The enduring focus on reducing FCR in livestock, even so, also led to large amounts of human-edible plant products, like cereal grains, in livestock diets. Annually, about 1 billion tons of cereal grains are fed to livestock (Eisler et al. 2014). Directly consumption of these cereals by humans is more efficient in terms of global land employ than consumption of products derived from livestock fed with these cereals because free energy is lost during conversion from plant to animal product (Goodland 1997). In a situation where state availability is no longer abundant, i.eastward. feeding a growing earth population with a given amount of land, improving FCR in livestock might not necessarily imply improving global land apply efficiency. To determine the role of livestock in terms of global food supply, we are in need of a measure that accounts for the contest for land between livestock and human.
One way to measure this competition for land is to compute human-edible protein and energy conversion ratios (Wilkinson 2011; Dijkstra et al. 2013). These conversion ratios represent the amount of energy or protein in animal feed that is potentially edible for humans over the corporeality of energy or poly peptide in that creature product that is edible for humans. Ratios above one, such as for UK broilers, laying hens, pigs and some cattle, are unsustainable because animals produce less edible protein and/or energy than they swallow (Wilkinson 2011). A ratio below 1, such as for UK milk production (Wilkinson 2011), does not immediately imply efficient land use in terms of global food supply considering these conversion ratios practise not yet include the fact that, for example, grass fed to dairy cows tin be produced on land suitable for the cultivation of human food crops, or in other words, they do not include the opportunity costs of state for human being nutrient production.
The to a higher place-described conversion ratios originally focused on the efficiency of animals to convert feed or specifically human-inedible feed into animal products. Besides improving crop yield per hectare or the feed efficiency of animals, it is increasingly recognized that land use efficiency by livestock should be examined along the entire livestock supply chain. Over the last years, several studies were published that assessed state apply by livestock along the entire supply chain (De Vries and De Boer 2010), generally using life cycle assessment (LCA). At present, LCA is an internationally acknowledged method to quantify employ of natural resources, such as land or fossil energy, during the entire life bike of a product (Guinée et al. 2002). An LCA quantifies the land needed to produce ane kg ASF and implicitly combines data nigh crop productivity (i.e. ingather yield per ha) and animal productivity (i.due east. feed efficiency forth the concatenation, including breeding, rearing and producing animals). Current LCA results show that production and utilization of feed are the dominant factors determining country use efficiency of livestock systems. Several LCA studies adamant the land use efficiency of contrasting livestock products. They concluded that product of 1 kg of beef protein uses almost state, followed by production of 1 kg of pork, craven, egg or milk protein (De Vries and De Boer 2010; Eshel et al. 2014). Interpretation of current LCA results, even so, is hindered because results practice not include differences in consumption of human-edible products by livestock or differences in suitability of land used for feed production to directly cultivate food crops, or in other words, they do not account for the competition between humans and animals for state. Grass-fed beef cattle, for example, generally swallow less human-edible products than pigs or poultry and can value grassland that is less suitable for product of food crops.
Several LCA studies did propose a mode to account for differences in quality of state (Ridoutt et al. 2012; Borucke et al. 2013). Net primary productivity of potential biomass (NPP0, one thousand C m−2 year−1), for instance, was used as proxy to account for differences in land quality (Ridoutt et al. 2012). According to this approach, land use of diverse agricultural products is assessed past multiplying each spatially differentiated area of land use by its internet chief productivity divided by global boilerplate net primary productivity. Using cyberspace master productivity equally a proxy for country quality, nonetheless, does non yet include the fact that, for example, feed crops fed to dairy can be produced on land less suitable for the tillage of human food crops or, in other words, that livestock can produce human-edible protein from state with depression opportunity costs for human being food production.
Existing measures for efficiency of country apply for livestock systems capture unlike aspects of the fence. The FCR focuses on the efficiency of animals to convert feed into beast products; poly peptide and energy conversion ratios focus on the efficiency of animals to catechumen homo-inedible feed into animal products; and an LCA focusses on the total amount of country required to produce 1 kg ASF and combines plant and animal productivity. None of these measures includes the opportunity costs of land for crop production. To address the contribution of livestock to increase nutrient supply and therefore contribute to food security, we are in need of a measure for land use efficiency that accounts for plant productivity, efficiency of converting especially human-inedible feed into animal production and the opportunity price of land for ingather cultivation and has a life cycle perspective.
Novel measure for land employ efficiency of livestock systems
Our measure out of land apply efficiency of livestock systems includes all in a higher place-mentioned aspects to determine the role of livestock in terms of food supply and is divers equally the post-obit state use ratio (LUR):
$$ \mathrm{L}\mathrm{U}\mathrm{R}=\frac{{\displaystyle {\sum}_{i=1}^n{\displaystyle {\sum}_{j=1}^m\left({\mathrm{LO}}_{ij}\times \mathrm{H}\mathrm{D}\mathrm{P}\kern0.5em {\mathrm{chiliad}}^{-2}\kern0.5em {\mathrm{y}}_j^{-ane}\right)}}}{\mathrm{HDP}\kern0.5em \mathrm{of}\kern0.5em \mathrm{i}\kern0.5em \mathrm{kg}\kern0.5em \mathrm{A}\mathrm{S}\mathrm{F}} $$
where LO ij is the land area occupied for a year (m2 year) to cultivate the amount of feed ingredient i (i = 1,due north) in state j (j = 1,k) that is needed to produce 1 kg ASF, including breeding and rearing of young stock, and HDP j is the maximum amount of human-digestible protein (HDP) that can be produced per m2 year by direct cultivation of food crops in country j. The denominator contains the amount of HDP of 1 kg ASF.
To compute the LUR of one kg ASF from a specific livestock organisation, 4 steps are required. Showtime, you quantify the land area occupied (LO ij ) to cultivate the amount of each feed ingredient (i = 1,due north) in the different countries of origin (j = 1,k) that are needed to produce ane kg ASF. Second, you lot assess the suitability of each land area occupied to straight abound man nutrient crops, using the crop suitability index (IIASA and FAO 2012). Third, for each area of country suitable for direct cultivation of food crops (LO ij ), you lot determine the maximum HDP j from cultivation of food crops past combining information almost crop yield per hectare for each suitable crop, with its protein content and homo digestibility. The amount of HDP that can be produced on all land required for feed production is summed and used as numerator. Quaternary, yous assess the corporeality of HDP in 1 kg ASF, which is the denominator.
LUR, therefore, represents the maximum amount of HDP derived from nutrient crops on all land used to cultivate feed required to produce 1 kg ASF over the corporeality of HDP in that i kg ASF. A ratio higher up one implies that the land required to produce this kilogramme ASF would yield more HDP if used directly to cultivate homo nutrient crops, whereas a ratio below 1 implies that livestock product is the best fashion to produce HDP from that land.
The iv steps of our concept will exist farther explained past computing the three example studies described beneath.
Computation of state employ efficiency of instance systems
To illustrate our concept, nosotros computed our novel method for three example systems in the Netherlands: production of ASF from laying hens (eggs and meat) and production of ASF from dairy cows (milk and meat from the dairy farm) on peat soils and on sandy soils. Nosotros distinguished dairy farming on peat soils and sandy soils because of their departure in suitability to cultivate food crops. Furthermore, we compared our novel method with currently bachelor methods; FCR, the protein and energy conversion ratio and LOLCA, for protein and energy. The calculations for our novel method and the current methods are based on the same data.
Land use ratio
- 1.
Quantify land area occupied to cultivate feed ingredients. We analysed the most common laying hen organization in holland, i.e. a multi-tier befouled system with brown hens. Our production arrangement included the rearing and laying hen stage, whereas production of day-quondam hens and parent stock were excluded. Feed intake of rearing and laying hens was calculated based on technical data of KWIN-V 2013 (Tabular array 1).
Table 1 Technical and economic data for Dutch egg production in a multi-tier barn system (KWIN-V 2013)
Rearing hens were assumed to eat just one type of concentrate feed. The composition of this concentrate feed was based on Dekker et al. (2011) and is reported in Table 2. Laying hens were fed a starter feed during the get-go 23 weeks, followed by a regular feed (personal communication, L. Start, Schothorst Feed Research, Lelystad, kingdom of the netherlands). The composition of starter and regular feed for laying hens was based on recent advices for commercial feed (Gijsberts 2013a, b, c, d). The weighted average of the starter and regular feed of laying hens is presented in Tabular array two.
For each feed ingredient, the state of origin and yields per hectare were based on a database called 'feedprint', feedprint (based on currently bachelor literature) provides information on the ecology impact of feed ingredients used in the netherlands (Vellinga et al. 2013). Given the exact amount of feed ingredients consumed, and their yields per hectare, nosotros quantified the surface area occupied to cultivate all feed ingredients. In case of a multiple-output situation, state use was allocated to the diverse outputs based on their relative economic value (i.east. economic allotment). Crop residues, such as citrus and beet lurid, maize gluten meal and straw, were assumed to accept an economic value of null (Vellinga et al. 2013).
For dairy farming, we selected dairy production systems in the Netherlands with >90 % sandy soils or >xc % peat soils. Technical data required to determine all land used to cultivate feed required to produce one kg ASF from this system were based on the Dutch Farm Accountancy Data Network (FADN 2014) and are shown in Tables iii and four. Feed intake of the whole dairy herd, including immature stock, was based on average technical data betwixt 2010 and 2012 (Tabular array 3).
Table 3 shows average technical and economic information for Dutch dairy production system on peat or sand. To quantify the land occupied for the cultivation of a feed ingredient, we corrected these data for feeding and conservation losses (Tabular array four). Feeding and conservation losses of grazing animals were assumed negligible (RIVM 2013). Furthermore, nosotros assumed all maize silage to be purchased because no data were available to exactly determine the production of on-farm maize silage.
Purchased concentrates were assumed to be seventy.5 % protein-rich (19.6 % crude protein) and 29.five % very protein-rich (thirty.4 % crude protein) for dairy cows on sandy soils, and 16.2 % standard (fourteen.one % crude protein) and 83.eight % protein-rich for dairy cows on peat soils (Table 5). Table 5 shows the limerick of each type of concentrate. The corporeality of both concentrate types purchased was computed by combining data from the total amount of crude protein and energy in purchased concentrates (Tabular array 3) and the rough protein-to-energy ratio of both types of concentrates (Vellinga et al. 2013). Given the amount of concentrates consumed, and their related average yields per hectare, we quantified the area occupied to cultivate all feed ingredients (Vellinga et al. 2013).
Similarly, the amount of purchased moisture by-products, brewers grain and sugar beet pulp was computed by combining information from the full amount of crude protein and free energy in purchased moisture by-products (Table 3) and the rough protein-to-free energy ratio of both products. Given the amount of feed ingredients consumed, and their yields per hectare, we quantified the area occupied to cultivate all feed ingredients. For on-subcontract feed product, yields per hectare were obtained from the Dutch Farm Accountancy Information Network (FADN 2014; Table iii). For crop production outside the farm, country of origin and yields per hectare were obtained from feedprint (Vellinga et al. 2013).
- two.
Determine the suitability of land to directly cultivate human nutrient crops. For each area of land identified for feed production, we determined its suitability to directly abound food crops. On each surface area of country suitable to abound food crops, dissimilar crops can be cultivated. Nosotros focused on the five major food crops as the yield of those crops are loftier and therefore the corporeality of poly peptide per hectare is also high. The suitability for the 5 major nutrient crops, i.due east. wheat (wetland and indica dry land), maize, potatoes (white and sweetness) and soybeans, at a specific location was assessed based on the Global Agro-Ecological Zones (GAEZ) database (IIASA and FAO 2012). This database classifies crop suitability in eight groups (varying from very loftier to non suitable), by quantifying to what extent soil (e.g. pH, soil water property capacity) and climatic weather condition (e.chiliad. wet 24-hour interval frequency, sunshine, temperature) lucifer crop requirements, under defined input and direction circumstances. We assessed ingather suitability for electric current cultivated land in a state of affairs of loftier input levels, optimal water supply and baseline climate conditions (1961–1990). If the suitability of the ingather was skilful, high, or very high (suitability index > 55), the land was considered suitable to cultivate that specific crop.
- 3.
Calculation of HDP production from all land suitable for ingather production (numerator). Human-digestible protein product from the five selected crops was calculated from their respective yields (Table half dozen), multiplied past their protein content and digestibility (Table 7). For on-subcontract land used for grass production, crop yields per hectare were assumed to be soil specific. Peat soils were assumed unsuitable for the tillage of any of the v major food crops, whereas sandy soils were assumed suitable for tillage of white potatoes (i.e. 56,000 kg/ha) and wheat (7300 kg/ha) (KWIN-AGV 2012). For off-farm crop production, state-average yield data from FAOstat were used (Table 6), as information nigh exact location and, consequently, soil type were missing. Afterward, the highest HDP for each surface area of land was chosen and summed across all state areas required to produce 1 kg of ASF. This sum of HDP was used as numerator of our land use ratio. Because animal-source nutrient contributes not only to the protein but too to the free energy demand of humans, we also computed our country employ ratio from an energy perspective, implying that HDP was replaced past man-digestible energy. For man-digestible free energy, values of the energy content (Table 7) were directly derived from a USDA database (Us Department of Agriculture (USDA) 2013). For chicken meat, nosotros causeless that 56 % of live weight was edible and for beefiness, this was 43 % (De Vries and De Boer 2010).
Tabular array vi Land average yields (kg/ha) of 5 major food crops for the yr 2011 (FAOstat; http://faostat.fao.org). An empty cell implies a country was considered unsuitable to cultivate that crop (i.e. suitability alphabetize < 55) Table vii Dry matter (DM), protein and homo-digestible (HD) energy contents of products and human digestibility value of poly peptide - 4.
Calculation of HDP of 1 kg of fauna-source food (denominator). The amount of HDP in 1 kg of ASF was computed by multiplying with its protein content and its poly peptide digestibility for humans. The corporeality of man-digestible energy in 1 kg of ASF was computed by multiplying with its free energy content for humans.
Assessing existing measures of land utilise efficiency of livestock systems
To demonstrate our concept, we compared LUR values with existing measures of country utilize efficiency. To permit an accurate comparison, nosotros computed existing measures (FCR, protein and energy conversion ratios, and LOLCA) for each instance system using the same information as nosotros used to compute LUR.
The feed conversion ratio (FCR) was divers every bit the corporeality of DM in feed supplied to the producing animal over the kilogramme of main output of that animal (kg egg and kg fat-poly peptide-corrected milk; CVB 2012). A higher FCR indicates a lower efficiency of converting feed into animal product and implies a lower efficiency of country use. Feed intake was already assessed in the first step of our novel approach. Feed intake data as presented in Tabular array 3, notwithstanding, were computed for the entire herd. To correct for feed intake of young stock in the ciphering of feed, energy and protein conversion ratios, we assumed a total intake from birth until get-go lactation of 31 GJ NEL per animal (NEL = net free energy for lactation), of which 19 % consisted of concentrate feed and milk products (CVB 2012; Dijkstra et al. 2013). All milk products equally presented in Table 3 were assumed to be consumed past immature stock. Boilerplate concentrate feed and roughage limerick was causeless to be like for young stock and producing cows per instance system. No wet past-products were assigned to young stock. Based on those assumptions, we calculated a total feed intake of dairy cows on sandy soils of 246 GJ NEL grass grazing, 1300 GJ NEL grass silage, 1346 GJ NEL maize silage, 1001 GJ NEL concentrate feed and 206 GJ NEL moisture by-products and dairy on peat soils of 491 GJ NEL grass grazing, 1590 GJ NEL grass silage, 421 GJ NEL maize silage, 902 GJ NE50 concentrate feed and 329 GJ NEL wet past-products. The DM content of feed ingredients was taken from feedprint (Vellinga et al. 2013), whereas the DM content of on-farm grass was taken from the Dutch Farm Accountancy Data Network (FADN 2014; Table 3).
The protein conversion ratios were defined as the ratio of rough protein in creature feed straight edible for humans over kilogramme protein in eggs or milk. Similarly, the energy conversion ratio was defined every bit the ratio of gross energy in animal feed directly edible for humans over kilogramme gross energy in eggs or milk. A ratio above 1 implies that an creature produces less edible poly peptide than information technology consumes and appears inefficient from a land-use perspective. The human being-edibility of feed products was taken from the literature (Wilkinson 2011), whereas ASF was considered to be fully human-edible. We used boilerplate food composition values for eggs (USDA 2013), whereas for milk, we used case-specific protein contents (Table 3).
Likewise examining feed and protein conversion ratios at animal level, we too computed country use associated with feed production forth the life wheel of one kg HDP from laying hens or dairy cows, including rearing of young stock. Land area occupied to cultivate feed ingredients along the chain was assessed already in the first footstep of our novel arroyo (meet previous paragraph). Subsequently, information technology was allocated to the main product of the livestock system (i.eastward. egg or milk) based on economic allocation and expressed per kilogramme of man-digestible protein or per kilogramme of human-digestible energy.
Results
We first present LUR values from a 'protein' perspective, equally livestock products contribute especially to the protein demand of humans (Galloway et al. 2007; De Vries and De Boer 2010) and compare those with results from existing measures of state apply efficiency. Second, nosotros present and compare results from an free energy perspective.
Results of land utilize ratio (protein perspective)
The LUR for the case of laying hens equaled 2.08. A LUR of 2.08 implies that the state required to produce 1 kg HDP from laying hens could straight yield two.08 kg HDP from human food crops. The structure of the ciphering of the LUR is depicted in Fig. one. To produce 1 kg of fresh egg and its associated production of 0.068 kg chicken meat, we needed 2.30 kg of feed for laying hens and 0.284 kg of feed for rearing hens. The main feed ingredients of laying hen feed were maize (54 %), soybean meal (17 %) and wheat (8 %). One-half of this maize was assumed to originate from Deutschland and the other half from French republic. With a yield of 8788 kg per hectare, production of 0.62 kg maize in Germany required 0.71 gtwo year. This 0.71 mii year could have been used directly to produce homo food crops and could yield maximally 0.049 kg HDP ((7019 kg wheat per ha × 0.904 DM per kg wheat × 125.1 yard protein per kg DM wheat × 87 % digestibility / 10,000) × 0.71 m2 twelvemonth). In total, the land used to produce 1 kg of eggs and associated chicken meat could accept yielded directly 0.27 kg of HDP from human being food crops. One kilogramme of eggs contains 0.12 kg HDP, whereas 0.07 kg of chicken meat contains 0.01 kg HDP. The LUR of eggs, therefore, equaled 0.27/0.13 = 2.08.
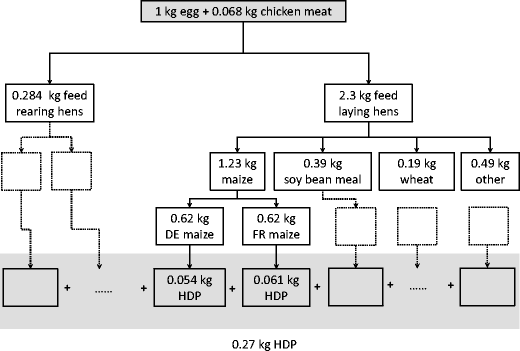
Analogy of our concept of land use ratio for the case system of laying hens, assuming a production of 1 kg of eggs and an associated product of 0.076 kg of chicken meat (DE Germany, FR France, HDP homo-digestible protein)
Similarly, we determined a LUR of two.ten for dairy cows on sandy soils and 0.67 for dairy cows on peat soils. The LUR of dairy cows on sand was similar to the LUR of hens, despite the fact that compared with the nutrition of laying hens, the diet of dairy cows independent less products that humans could swallow directly (i.e. 72 % of rough protein in diets of laying hens was human-edible compared to 16 % for dairy cows on sand and 9 % for dairy cows on peat). The country used to produce feed ingredients for laying hens and dairy cows on sandy soils, nonetheless, appeared to have about the aforementioned potential to directly produce HDP past nutrient crops. This was not the case for dairy cows on peat soils. The land used to grow grass and grass silage for these dairy cows was causeless unsuitable for direct product of food crops, overall resulting in a LUR of 0.67. This LUR implies that the land required to produce 1 kg HDP from dairy cows on peat soils could only yield 0.67 kg of HDP from human nutrient crops straight. A LUR <i.0, therefore, is considered efficient in terms of global nutrient supply and implies that animals produce more HDP per square metre than crops. Values <i.0 demonstrate that livestock practice contribute to food supply and therefore food security. Our LUR offers identification of livestock production systems that use state efficiently in terms of nutrient supply. Country-efficient livestock systems typically value land with low opportunity costs for arable production (east.grand. peat soils or wet grasslands) and/or by-products from crop tillage or the food or energy industry (e.thousand. beet lurid).
Results of existing measures of state use efficiency of livestock systems
The FCR for producing eggs is roughly twice as high as the production of milk on peat (Fig. two), which is in line with a U.k. case study (Wilkinson 2011). The FCR of dairy cows on sandy soils, however, was virtually 15 % lower than the FCR of dairy cows on peat soils. Differences in FCR are determined mainly by differences in annual milk production per cow (Dijkstra et al. 2013). Almanac milk production per cow indeed was comparable for Dutch cows on peat (i.e. 6350 kg) and cows in the U.k. case study (Dijkstra et al. 2013) (6500 kg) but was higher for dairy cows on sand (8114 kg). Based on this definition of FCR, nosotros would conclude that dairy cows are more than efficient than laying hens, whereas dairy cows on sand are nigh efficient. Relative to eggs, however, milk has a lower DM content (i.e. milk = 12.three % DM; eggs = 23.nine % DM; (18)). When we express FCR as kg DM in feed over kg DM in production, differences amongst FCRs betwixt milk product and egg production are less pronounced (FCRegg = eight.half dozen; FCRmilk sand = 6.three; FCRmilk peat = 7.4), which is in agreement with Galloway et al. (2007).
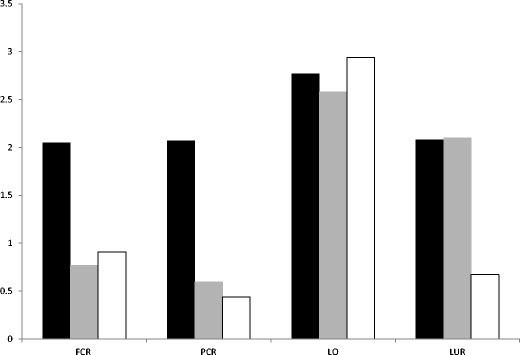
Feed conversion ratios (FCR) in kg dm/kg product (i.e. eggs or milk), poly peptide conversion ratios (PCR) in kg human-edible protein/kg human-edible product, life bike assessments of land occupation (LO) in ten gii year/kg human-digestible protein product and our newly adult land use ratio (LUR) in kg human-digestible protein in crops/human-digestible protein in animal source food. All methods are applied for 3 case systems in the Netherlands, laying hens (black), dairy cows on sand (grey) and dairy cows on peat (white)
The protein conversion ratios of laying hens are higher compared to dairy cows, which is in the range with results in literature (Wilkinson 2011; Dijkstra et al. 2013). Our laying hen organization shows a protein conversion ratios of about 2. In terms of global food supply, therefore, the existing manner of egg production is not country efficient. A target ratio beneath i may be possible by replacing, for example, cereals or soybean meal (both have a loftier proportion of edible protein) by waste-fed insects or by by-products from the food or free energy industry with a low economic value. Our dairy systems show a protein conversion ratios <1.0, clearly demonstrating the ability of ruminants to turn human-inedible feed ingredients into man-edible product. The protein conversion ratios of dairy cows on peat was lower (0.44) than of cows on sand (0.60) considering cows on peat consume relatively more grass. Grass has a relatively high protein content. Concentrates fed to dairy cows on peat, therefore, have a lower poly peptide content than concentrates fed to cows on sand. In contrast to grass, some ingredients in concentrates are human being-edible. The difference in protein conversion ratios between cows on peat and sand, therefore, is explained past the difference in protein content of concentrates fed to cows on peat and sand. Based on the protein conversion ratio results, we would conclude that dairy cows are more than efficient than laying hens, whereas dairy cows on peat are almost efficient.
Land utilise for production of one kg HDP from laying hens required slightly more compared to the production of i kg HDP from dairy cows on sandy soils but slightly less compared to the product of 1 kg HDP dairy cows on peat soils. Based on these Dutch case studies, therefore, we would not conclude that dairy production is more efficient than egg product. Moreover, production of milk on peat soils appears least efficient.
Results from an 'energy' perspective
Besides protein, ASF in many parts of the world also contributes to the free energy demand of humans. For existing measures of land use efficiency, the chief conclusions from the comparison amongst livestock systems presented in this study are valid also when presented from the 'energy' perspective, albeit slightly numerically modified (Table 8). Using human being-digestible energy instead of HDP in our computation of LUR, however, yielded different results: 6.39 for laying hens, ane.22 for dairy cows on peat soils, and 4.35 for dairy cows on sandy soils. These results demonstrate that none of our Dutch case systems produced human-digestible energy more efficiently than crops and back up earlier findings that plants produce free energy more than efficiently than poly peptide (Penning De Vries et al. 1974), whereas for livestock, this is reversed (Phuong et al. 2013). From the perspective of food supply, therefore, the main role of livestock in a human diet is provision of protein.
General discussion
The fact that livestock especially contribute to the protein demand of humans justifies our choice for HDP in the LUR. Protein digestibility was taken into business relationship to right for differences in protein quality between institute and animal products, whereas differences in essential amino acid content between plants and animals were not accounted for. Besides protein, ASF has other nutritional qualities, such as the provision of atomic number 26 and vitamin B12. In principle, we could extend our LUR not only to consider man-digestible poly peptide just also to include, for example, available iron, calcium, essential amino acids or vitamins. This, however, would crave an index for nutritional quality of a food detail. Such indices have been adult for private food items and are referred to as nutrient density scores. These density scores relate the nutrient content of 100 g or 100 kcal of a product to the daily recommended intake and average the values of different nutrients into one final score (Drewnowski and Fulgoni 2014). Despite a low nutrient density score, however, an individual food item tin be valuable at the dietary level because of its richness in one very scarce nutrient. We believe, therefore, that the full range of nutritional needs should be met at the level of the unabridged human diet.
By applying the LUR method, information technology is possible to increase country use efficiency. However, to identify the contribution of livestock to future sustainable diets, i should also appraise the contribution of livestock systems to global warming, acidification, eutrophication, water use, biodiversity and other ecology impacts. For example, a ruminant system on marginal grassland with a LUR < i might accept a relatively high global warming potential as feeding gristly diets increases the production of enteric marsh gas simply could also contribute to the preservation and enhancement of biodiversity and the conservation of cultural landscapes when grasslands are managed well. We demonstrated the LUR for three case systems: laying hens, dairy cows on sandy soils and dairy cows on peat soils. These case systems represented existing livestock systems and were deliberately chosen considering literature showed that they had comparable land use requirements from a life bike perspective, while they differed in the percentage of human-edible feed in diets of animals and in opportunity costs of state for ingather tillage (sandy versus peat soils). Moreover, ciphering of LUR requires global, high-resolution inventory information, which were partly bachelor for these existing systems. Despite the data availability, the LUR guess of our case systems could have been refined further if the exact production location and associated yields of all purchased feed ingredients would have been known. Such detailed information about feed ingredients, all the same, is generally absent.
Our concept is applicable to a large variety of livestock systems and is of paramount importance in the debate about the part of livestock in global food supply. Several, especially LCA-based studies recommended to switch from an animal-based to a plant-based homo nutrition, whereas others communication to substitute beef by pork or chicken to minimize land use in an beast-based diet (Stehfest et al. 2009; Meier and Christen 2012; Eshel et al. 2014). The above-mentioned studies, all the same, do not account for differences in the suitability of land to directly produce food crops. Our results clearly demonstrate that ruminant systems that value land with low opportunity costs for arable production tin produce HDP more efficiently than crops and, therefore, exercise accept a function in hereafter nutrient supply. In a state of affairs of land scarcity, therefore, a plant-based nutrition is non more than land efficient than a nutrition including beast-source food from, for example, ruminants grazing on country less suitable for crop production. Analogously, simply substituting beef past pork or chicken does not automatically imply improving efficiency of land employ in terms of food supply. Beefiness and/or milk produced from grass on peat soils just would even effect in a LUR of cypher.
Our LUR enables identification of country-efficient livestock systems and allows further comeback of systems regarding efficiency of land employ to contribute to futurity nutrient supply. Land-efficient livestock systems (i.eastward. LUR < 1.0) typically value land with low opportunity costs for arable production (e.chiliad. beef or dairy cattle grazing 'marginal land') and/or by-products from food or bio-energy production (eastward.g. pigs eating beet pulp or rapeseed meal). The amount of ASF that can exist produced from 'marginal land' and by-products might not be sufficient to feed each human being being a Western European or American diet. We acknowledge, therefore, that improving land use efficiency of livestock systems implies a more modest consumption of ASF in affluent countries. In countries where dietary diverseness is limited and malnutrition levels are high, withal, an increase in consumption of ASF is legitimate. A modest consumption of ASF is required also to temper ecology impacts of current and expected future demands of ASF. To use country efficiently, therefore, we should aim at increasing livestock productivity while maintaining a LUR < one.0.
Conclusions
Our results demonstrate that existing measures for efficiency of land use for livestock systems requite insight into different aspects of the contend well-nigh the contribution of livestock to food supply and therefore food security. Conversion ratios are used to gain insight into the power of animals to convert feed, or more specifically human-inedible feed, into beast products. Results show that improving the conversion of man-inedible feed into beast product improves land efficiency merely if feed is produced on land with depression opportunity costs for arable production (i.east. protein conversion ratios is lower for dairy cows than for laying hens, whereas LUR is similar for dairy cows on sandy soils and for laying hens). LCA results are used to gain insight into the country required to produce 1 kg ASF along the entire chain. Land apply per kilogramme ASF reduces by increasing crop yield per hectare, reducing the feed conversion ratio and increasing the reproductive performance of animals. Country requirements per kilogramme ASF for cows on sand indeed are lower than for cows on peat, mainly because of the reduced FCR. This reduced FCR, however, as well implied a diet with more human-edible plant products, i.e. the protein conversion ratios of cows on sand indeed was higher than of cows on peat. Improving LCA results of land use, therefore, might indirectly increase the amount of man-edible feed in diets of livestock and, every bit such, reduce the efficiency of country use in terms of food supply. None of the in a higher place-mentioned measures accounts for the opportunity costs of state to cultivate human being food crops. Our LUR includes all aspects of importance to determine the role of livestock for future food supply, and, therefore, yields a more complete insight into land employ efficiency for livestock systems. Results demonstrated that ruminant systems that value land with low opportunity costs for arable production tin can produce HDP more than efficiently than crops and, therefore, do have a role in future food supply. Values <1.0 demonstrate that livestock exercise contribute to food supply and therefore to food security. Our LUR offers identification of livestock product systems that apply state efficiently in terms of nutrient supply. Land-efficient livestock systems typically value land with low opportunity costs for arable product (e.g. peat soils or wet grasslands) and/or by-products from crop tillage or the food or free energy manufacture (eastward.g. beet lurid).
References
-
Borucke M, Moore D, Cranston G, Gracey K, Iha K, Larson J, Lazarus E, Morales JC, Wackernagel M, Galli A (2013) Bookkeeping for demand and supply of the biosphere's regenerative capacity: the National Footprint Accounts' underlying methodology and framework. Ecol Indic 24:518–533
-
CVB (2012) Feed tables. (in Dutch: Tabellenboek veevoeding. CVB-reeks nr. 50.) Productschap Diervoeder, Centraal Veevoederbureau, the Haque, the Netherlands
-
De Vries M, De Boer IJM (2010) Comparing environmental impacts for livestock products: a review of life cycle assessments. Livest Sci 128(i–3):1–xi
-
Dekker SEM, De Boer IJM, Vermeij I, Aarnink AJA, Groot Koerkamp PWG (2011) Ecological and economic evaluation of Dutch egg production systems. Livest Sci 13(1–2):109–121
-
Dijkstra J, France J, Ellis JL, Strathe AB, Kebreab E, Bannink A (2013) Productions efficiency of ruminants: feed, nitrogen and methyl hydride. In: Kebreab Due east (ed) Sustainable brute agriculture. CAB International, Wallingford, pp x–25
-
Drewnowski A, Fulgoni VL (2014) Nutrient density: principles and evaluation tools. Am J Clin Nutr 99(5):1223S–1228S
-
Eisler MC, Lee MRF, Tarlton JF, Martin GB, Beddington J, Dungait JAJ, Greathead H, Liu J, Mathew South, Miller H, Misselbrook T, Murray P, Vinod VK, Van Saun R, Wintertime M (2014) Steps to sustainable livestock. Nature 507:32–34
-
Eppendorfer WH, Eggum BO, Bille SW (1979) Nutritive value of potato crude protein equally influenced by manuring and amino acid composition. J Sci Food Agric 30(iv):361–368
-
Eshel G, Shepon A, Makov T, Milo R (2014) Land, irrigation water, greenhouse gas, and reactive nitrogen burdens of meat, eggs, and dairy production in the The states. Proc Natl Acad Sci 111(33):11996–12001
-
FAO (2009) The state of food and agriculture. Livestock in balance. FAO, Rome
-
Farm Accountancy Data Network (2014) Subset of dairy farms derived from the Dutch Farm Accountancy Data Network for the years 2010–2012. LEI Wageningen UR, the Hague
-
Foley JA, Ramankutty N, Brauman KA, Cassidy ES, Gerber JS, Johnston M, Mueller ND, O'Connell C, Ray DK, W PC, Balzer C, Bennett EM, Carpenter SR, Loma J, Monfreda C, Polasky South, Rockstrom J, Sheehan J, Siebert Due south, Tilman D, Zaks DPM (2011) Solutions for a cultivated planet. Nature 478(7369):337–342
-
Gahlawat P, Sehgal S (1998) Poly peptide and starch digestibilities and mineral availability of products developed from white potato, soy and corn flour. Institute Foods Hum Nutr 52(2):151–160
-
Galloway JN, Burke M, Bradford GE, Naylor R, Falcon Westward, Chapagain AK, Gaskell JC, McCullough East, Mooney HA, Oleson KLL, Steinfeld H, Wassenaar T, Smil V (2007) International merchandise in meat: the tip of the pork chop. Ambio 36(8):622–629
-
Garnett T (2009) Livestock-related greenhouse gas emissions: impacts and options for policy makers. Environ Sci Technol 12(four):491–503
-
Gijsberts K (2013a) Linear programming cattle, squealer, and poultry feeds—report no. 3, March 2013 (In Dutch: Lineaire programmering rundvee-, varkens en pluimveevoeders—Verslag nr. three, maart 2013). Schothorst Feed Research, Lelystad, the Netherlands
-
Gijsberts K (2013b) Linear programming cattle, pig, and poultry feeds—report no. 6, June 2013 (In Dutch: Lineaire programmering rundvee-, varkens en pluimveevoeders—Verslag nr. half dozen, juni 2013). Schothorst Feed Research, Lelystad, the Netherlands
-
Gijsberts K (2013c) Linear programming cattle, hog, and poultry feeds—study no. 9, October 2013 (In Dutch: Lineaire programmering rundvee-, varkens en pluimveevoeders—Verslag nr. ix, oktober 2013). Schothorst Feed Research, Lelystad, holland
-
Gijsberts K (2013d) Linear programming cattle, pig, and poultry feeds—report no. 12, January 2013 (In Dutch: Lineaire programmering rundvee-, varkens en pluimveevoeders—Verslag nr. 12, januari 2013). Schothorst Feed Research, Lelystad, the Netherlands
-
Gilani GS, Cockell KA, Sepehr E (2005) Effects of antinutritional factors on protein digestibility and amino acid availability in foods. J AOAC Int 88(3):967–987
-
Godfray HCJ, Beddington JR, Crute IR, Haddad L, Lawrence D, Muir JF, Pretty J, Robinson S, Thomas SM, Toulmin C (2010) Food security: the challenge of feeding 9 billion people. Scientific discipline 327(5967):812–818
-
Goodland R (1997) Environmental sustainability in agriculture: diet matters. Ecol Econ 23(3):189–200
-
Grassini P, Eskridge KM, Cassman KG (2013) Distinguishing between yield advances and yield plateaus in historical ingather production trends. Nat Commun 4, art. no. 2918
-
Guinée JB, Gorrée Thousand, Heijungs R, Huppes K, Kleijn R, De Koning A, Van Oers Fifty, Wegener Sleeswijk A, Suh S, de Haes HA U, De Bruijn H, Van Duin R, Huijbregts MAJ (2002) Handbook on life cycle cess. Operational guide to the ISO standards. Kluwer Academic Publishers, Dordrecht
-
IIASA, FAO (2012) Global Agro-ecological Zones (GAEZ v3.0). IIASA, Laxenburg, Republic of austria and FAO, Rome, Italy
-
Khan MA, Saeeda J, Rana IA, Ihsan U (1992) Improvement of wheat protein by supplementation with potato flour. Pak J Agric Res 13(2):101–106
-
Kies C, Fox HM (1972) Effect of amino acid supplementation of dehydrated potato flakes on poly peptide nutritive value for human adults. J Food Sci 37(iii):378–380
-
KWIN-AGV (2012) Quantitative information arable farming and open-air horticulture 2012 (In Dutch: Kwantitatieve Informatie Akkerbouw en Vollegrondsgroenteteelt 2012). Wageningen UR Praktijkonderzoek Institute en Omgeving, Lelystad, the Netherlands
-
KWIN-V (2013) Quantitative information livestock 2013–2014 (In Dutch: Kwantitatieve Informatie Veehouderij 2013–2014). Wageningen UR Livestock Research, Lelystad, holland
-
Meier T, Christen O (2012) Environmental impacts of dietary recommendations and dietary styles: Deutschland every bit an instance. Environ Sci Technol 47(2):877–888
-
Neeteson-van Nieuwenhoven A-M, Knap P, Avendaño South (2013) The role of sustainable commercial pig and poultry breeding for food security. Anim Front end iii(one):52–57
-
Penning De Vries FWT, Brunsting AHM, Van Laar HH (1974) Products, requirements and efficiency of biosynthesis a quantitative arroyo. J Theor Biol 45(2):339–377
-
Phuong HN, Friggens NC, de Boer IJM, Schmidely P (2013) Factors affecting energy and nitrogen efficiency of dairy cows: a meta-analysis. J Dairy Sci 96(eleven):7245–7259
-
Ravindran 5, Ravindran G, Sivakanesan R, Rajaguru SB (1995) Biochemical and nutritional assessment of tubers from xvi cultivars of sweetness potato (Ipomoea batatas Fifty.). J Agric Nutrient Chem 43(x):2646–2651
-
Ridoutt BG, Page G, Opie Grand, Huang J, Bellotti W (2012) Assessing carbon, water and land utilize footprints for beef cattle production in southern Australia. In: Corson MS, Van der Werf HMG (eds) eighth International conference on life cycle assessment in the Agri-Food Sector (LCA Nutrient 2012), i–iv October 2012, Saint Malo, France, ane–4 October 2012. INRA, Rennes, pp 599–604
-
RIVM (2013) Agricultural practise and water quality at grassland farms under derogation—results for 2011 inside the framework of the derogation monitoring network (in Dutch: Landbouwpraktijk en waterkwaliteit op landbouwbedrijven aangemeld voor derogatie–Resultaten meetjaar 2011 in het derogatiemeetnet). Vol RIVM Rapport 680717034. Bilthoven, the netherlands
-
Stehfest East, Bouwman L, van Vuuren DP, den Elzen MGJ, Eickhout B, Kabat P (2009) Climate benefits of changing diet. Clim Chang 95(1–2):83–102
-
Tilman D, Balzer C, Hill J, Befort BL (2011) Global food demand and the sustainable intensification of agriculture. Proc Natl Acad Sci U Southward A 108(50):20260–20264
-
USDA (2013) Limerick of foods raw, processed, prepared USDA national nutrient database for standard reference, release 26–documentation and user guide. U.S. Section of Agriculture—Agricultural Research Service, Beltsville
-
Vellinga TV, Blonk H, Marinussen M, Van Zeist WJ, De Boer IJM (2013) Methodology used in feedprint: a tool quantifying greenhouse gas emissions of feed production and utilization. Wageningen UR, Lelystad
-
Wilkinson JM (2011) Re-defining efficiency of feed use past livestock. Fauna v(07):1014–1022
Acknowledgments
We would like to give thanks Mark Dolman for providing necessary data for the dairy farming systems on sandy and peat soils and Theo Viets for his assist related to using software. We wish to thank Mike Grossman for his aid in formulating the title.
Author data
Authors and Affiliations
Respective author
Additional information
Responsible editor: Greg Thoma
Rights and permissions
Open Access This article is distributed under the terms of the Creative Commons Attribution 4.0 International License (http://creativecommons.org/licenses/past/iv.0/), which permits unrestricted apply, distribution, and reproduction in whatever medium, provided you give advisable credit to the original author(s) and the source, provide a link to the Artistic Eatables license, and point if changes were made.
Reprints and Permissions
Nearly this article
Cite this article
van Zanten, H.H.E., Mollenhorst, H., Klootwijk, C.West. et al. Global nutrient supply: land apply efficiency of livestock systems. Int J Life Bike Appraise 21, 747–758 (2016). https://doi.org/10.1007/s11367-015-0944-1
-
Received:
-
Accepted:
-
Published:
-
Issue Date:
-
DOI : https://doi.org/10.1007/s11367-015-0944-one
Keywords
- Feed efficiency
- Food security
- Human being-inedible feed
- State scarcity
- Life bike assessment
- Livestock
Source: https://link.springer.com/article/10.1007/s11367-015-0944-1
Posted by: bruggemaninden1957.blogspot.com
0 Response to "What Kinds Of Animals Are Most Efficient To Eat"
Post a Comment